Phase II – Achievements
Phase II – Achievements
The scientific outcome of the second funding period was extraordinarily fruitful surpassing even that of the first funding period. Many major achievements along the proposed research themes have been accomplished in and among all research areas of the SFB 953. Significantly, the level of interdisciplinary collaboration between the PIs spanning the fields of synthesis, physical characterization, device processing, and theory (quantum mechanical calculations) is very high. This, in turn, guaranteed the in-depth investigation of many fundamental, but also more applied topics in the field of synthetic carbon allotropes. Within the last years, the Friedrich-Alexander University of Erlangen-Nürnberg (FAU) was recognized as the leading carbon center worldwide. The funding instrument of the SFB 953 provides the organizational und financial basis for this success.
We have achieved fundamental breakthroughs with the systematic and comparative exploration of reductive synthesis protocols. For this purpose, we have activated graphene, carbon nanotubes, and fullerenes with alkali metals and studied the resulting intercalation compounds AMnSCA (AM = Li, Na, K) with respect to their physical and chemical properties such as an increased reactivity towards covalent addend binding. For example, we carried out the first in situ Raman monitoring of the reaction of graphite intercalation compounds with small electrophilic molecules exposed as gases. This allowed for the unequivocal assignment and resolution of individual lattice vibrational – Raman – modes of covalently functionalized graphene adducts. The evolution of these bands with increasing degree of functionalization from low to moderate levels provides a basis for the deconvolution of different components towards quantifying the extend of functionalization. By complementary DFT calculations, we were able to identify the vibrational changes in close proximity of the addend-bearing lattice C atoms and assign them to specific Raman modes. A particularly exciting discovery of the second funding period was the quantitative discharging of reduced graphite forms, such as graphite intercalation compounds (GICs), graphenide dispersions, and graphenides deposited on surfaces with benzonitrile applied as a simple liquid. Because of its relatively low reduction potential, benzonitrile is reduced during this process to the radical anion, which exhibits a red color and therefore serves as a reporter molecule for the quantitative determination of the amount of negative charges originally applied on the carbon sheets. Moreover, this discovery reveals a very fundamental physical-chemical phenomenon, namely, a quantitative solvent reduction induced and electrostatically driven mass transport of K+ ions from the solid GIC to the liquid phase. The simple treatment of dispersed graphenides suspended on silica substrates with benzonitrile also leads to a clean conversion to graphene. This unprecedented procedure represents a rather mild, scalable, and inexpensive method for graphene production surpassing previous wet-chemical processes.
We have pushed forward the general understanding of topochemical principles inherent to covalent graphene functionalization. For the first time, we studied the bis-functionalization of graphene by employing two successive reduction and covalent bond-forming steps. Bulk functionalization in dispersion and functionalization of individual sheets deposited on surfaces was compared. Whereas in the former case attacks from both sides of the plane are possible and can lead to strain-free architectures, in the latter case, retro-functionalizations are seen complementing earlier observations in fullerene and carbon nanotube chemistry. Another fundamental discovery was the experimental and theoretical proof that monolayer graphene is considerably more reactive than bilayer graphene. Supported by DFT calculations, we demonstrated that ditopic addend binding leads to much more stable products than the corresponding monotopic reactions. This is due to the fact that the subsequent monotopic (supratopic) additions to adjacent lattice C atoms lead to highly strained adducts whereas ditopic (antaratopic) additions can lead to completely strain-free situations (e.g., 2D diamond). This is an unprecedented and fundamental insight to graphene chemistry. Moreover, we were able to demonstrated that the preferred addition mode of graphene functionalization proceeds via all-trans hexaadditions to six-membered rings of the graphene lattice-forming perhydro-benzene-holes. These are generated either at rim-defects (graphene edges) or defects in the basal plane. Once a perhydro-benzene-holes is formed, further growth proceeds via perhydro-naphthalene-holes, perhydro-phenanthrene-holes, and so on. We name this process perhydro-PAH-annulations (PAH: polycyclic aromatic hydrocarbons). Two-dimensional disk-like growth is favored over linear growth. As a consequence, continuous growth patterns are generated starting from defects. These patterns contain rims or disk-like islands of per-functionalized graphane (2D diamond, sp3 regions) located next to intact sp2 graphene regions.
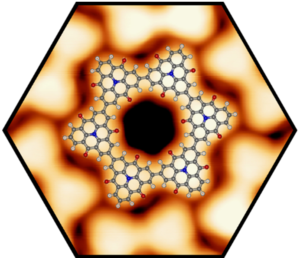
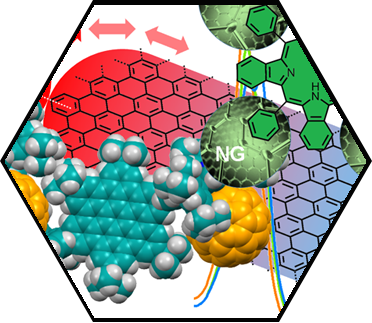
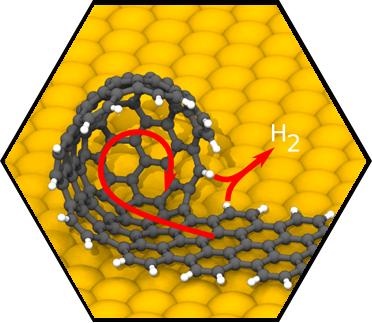
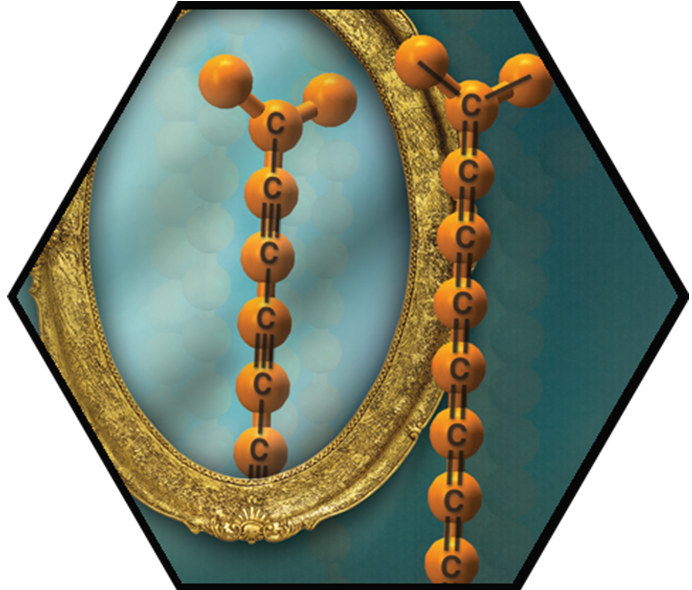
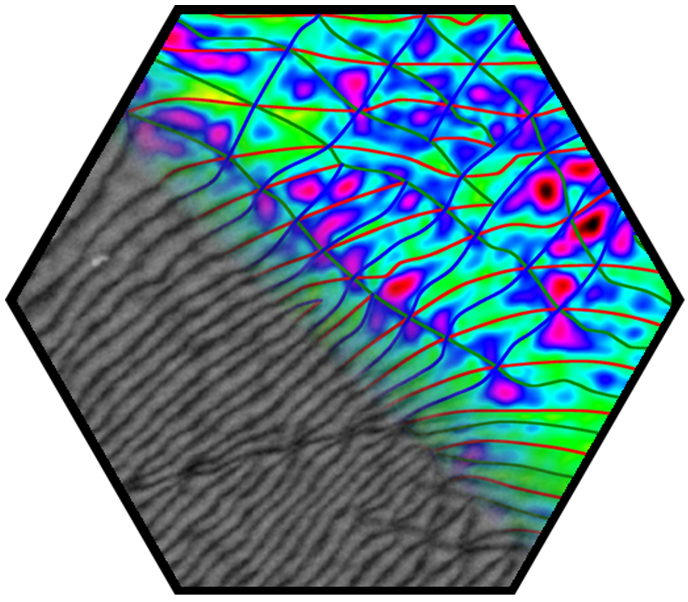
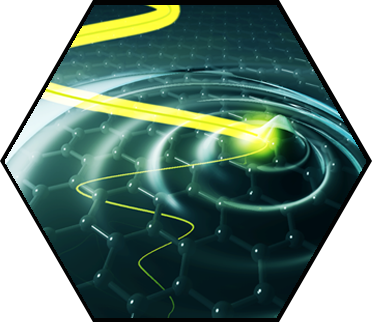
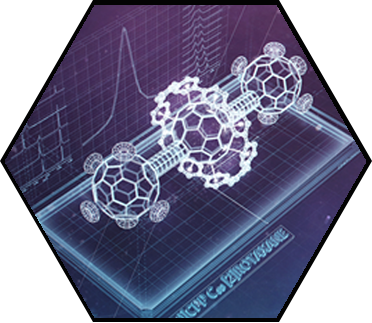
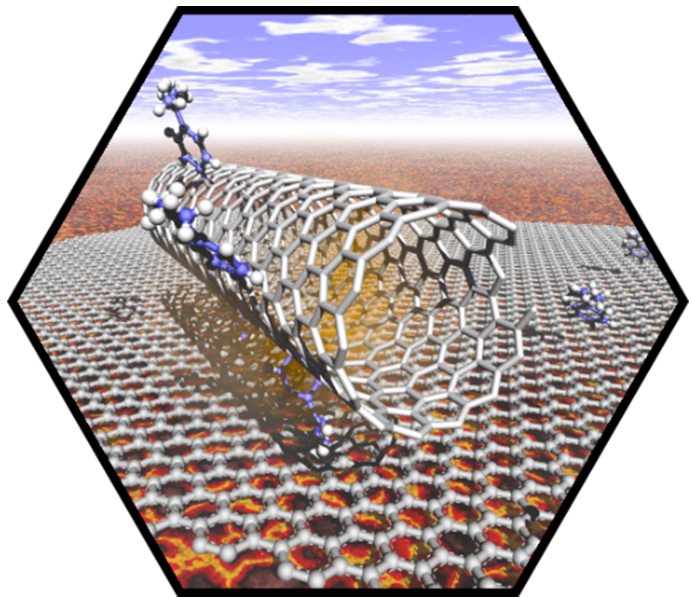